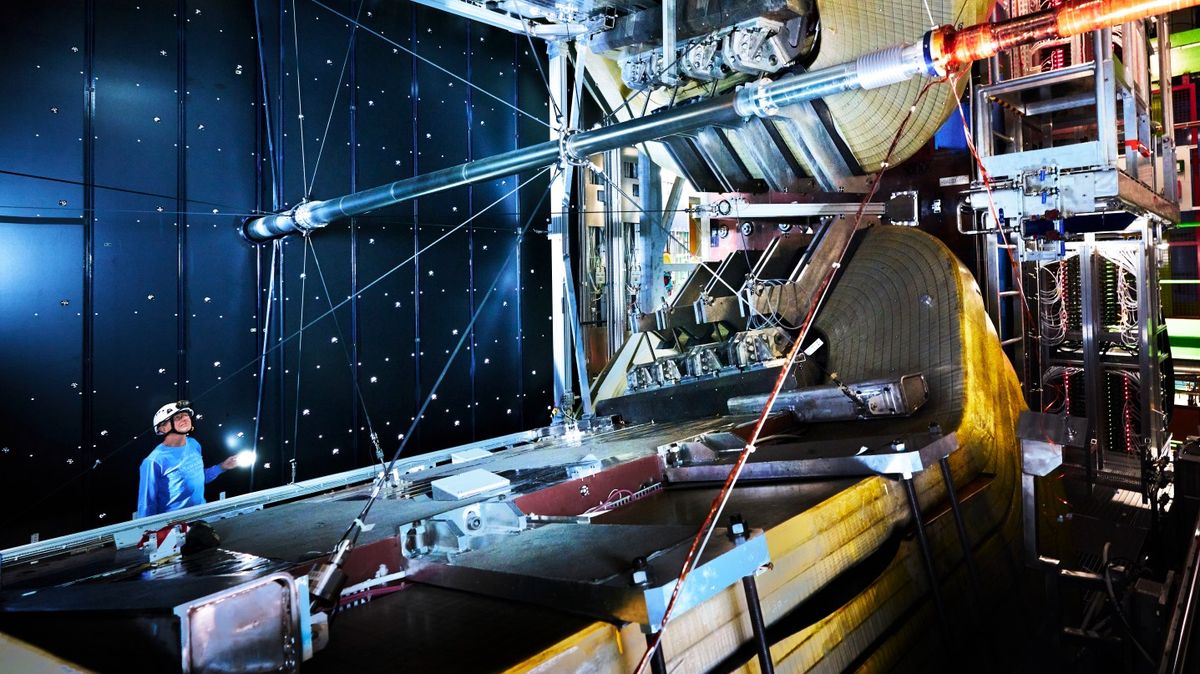
Physicists at the Large Hadron Collider (LHC) are closing in on an explanation for why we live in a universe of matter and not antimatter.
Matter and antimatter are two sides of the same coin. Every type of particle has an anti-particle, which is its equal and opposite. For instance, the antimatter equivalent of a negatively charged electron is a positively charged positron.
The Standard Model of physics tells us that if we substitute a particle for its antiparticle, it should still operate within the laws of physics in the same way. As such, the Big Bang should not have had a preference for creating one type over another — this symmetry at the heart of nature means that matter and antimatter should have formed in equal amounts in the Big Bang.
Related: 10 cosmic mysteries the Large Hadron Collider could unravel
Lucky for us, this does not seem to have been the case, because when you put matter and antimatter together, the outcome is explosive to say the least. Had matter and antimatter been crafted in equal amounts, then they would have annihilated each other, creating a cosmos filled with a sea of radiation, no atoms and no life. Today, the only antimatter is that which is produced in particle decays and interactions.
However, physicists still don’t have an explanation for why we are so fortunate. The fact that there’s an excess of matter in the universe means that, somewhere along the line, the symmetry in the way that matter and antimatter interact with the laws of physics was broken.
Physicists call this symmetry-breaking a “charge-conjugation parity (CP) violation.” One way to envisage it is to consider the rotational symmetry of a particle. Quantum physics theory holds that particles are not solid objects but rather strange little bodies that act like waves along a “wave function.” Ordinarily, when you spin that wave function around 360 degrees, the properties of the particle should not change. But when there is a CP violation, the properties of some particles can change — for instance, their quantum spin can alter from 1/2 to –1/2.
CP violation is known to take place in the weak force, which is the fundamental force that is responsible for radioactive decay inside atoms, so we know it can happen (although the weak-force example is a different CP violation than the one that could have possibly created the matter–antimatter imbalance). However, in 2013, scientists working on the LHCb (LHC–beauty) experiment also detected CP violation in the decay of “beauty mesons” and “strange beauty mesons,” in which the matter and antimatter versions of these particles behave differently when they decay.
The atoms in our bodies are made of protons and neutrons, which themselves are made of three smaller particles called quarks. Physicists call particles made of three quarks “baryons.” Particles made of two quarks (one quark and one anti-quark) are called “mesons,” and they tend to decay quickly. “Beauty” is another name for the “bottom” quark, while strange refers to a “strange” quark. (The names are just for descriptive purposes to differentiate quarks with slightly different properties and are not to be taken literally.)
Now, analysis of new and more comprehensive results from the LHCb experiment has measured more precisely than ever before the two most important parameters in the CP-violating decay of these mesons.
“These are key parameters that aid our search for unknown effects from beyond our current theory,” said LHCb spokesperson Chris Parkes in a statement.
Probing the decay of approximately 349,000 mesons, the LHCb team measured the angle at which the particles that come from the decay of the mesons were emitted, and the time taken for the mesons to decay. Both properties vary, depending on whether the meson is a matter or antimatter particle.
In particular, the time taken for a meson to decay (which is on the scale of tenths of a nanosecond) is dependent on the quantum state of the meson.
RELATED STORIES:
Experiments have observed that mesons are able to oscillate between their matter and antimatter states, which have ever-so-slightly different masses. This is because mesons exist in a state of “mixing:” they are a mixture of their matter and antimatter states, which allows them to oscillate back and forth between those states.
As the oscillations take place, the wave functions of the two states can interfere with one another, a bit like the constructive/destructive interference of light in the famous double-slit experiment. The time to decay depends strongly on the masses of the quantum states and the amount of interference between them, which results in a characteristic pattern of CP violation in the meson decays.
“These measurements are interpreted within our fundamental theory of particle physics, the Standard Model, improving the precision with which we can determine the difference between the behavior of matter and antimatter,” said Parkes. “Through more precise measurements, large improvements have been made in our knowledge.”
The LHCb team was able to measure these properties with unprecedented accuracy. Although the decay of mesons will not fully answer why there is more matter than antimatter in the universe, understanding the symmetry-breaking CP violation at the heart of their decays will help constrain models that do attempt to explain this strange asymmetry, which acted in force at the beginning of time to create a universe dominated by matter.
Follow Keith Cooper on Twitter @21stCenturySETI. Follow us on Twitter @Spacedotcom or on Facebook.